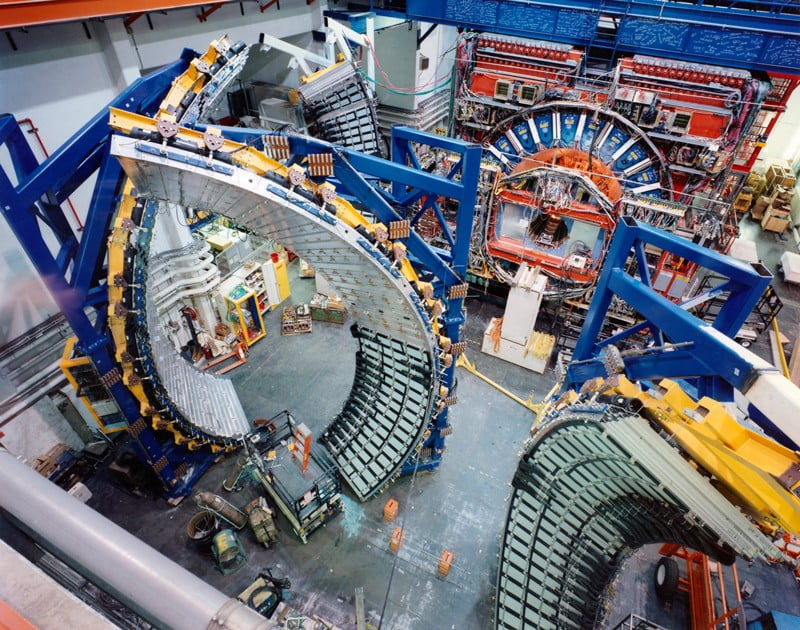
Particle’s surprise mass threatens to upend the standard model
- April 8, 2022
- 0
From its resting place outside Chicago, Illinois, a long-defunct experiment is threatening to throw the field of elementary particles off balance. Physicists have toiled for ten years to squeeze a crucial new measurement out of the experiment’s old data, and the results are now in. The team has found that the W boson — a fundamental particle that carries the weak nuclear force — is significantly heavier than theory predicts.
Although the difference between the theoretical prediction and experimental value is only 0.09%, it is significantly larger than the result’s error margins, which are less than 0.01%. The finding also disagrees with some other measurements of the mass. The collaboration that ran the latest experiment, called CDF at the Fermi National Accelerator Laboratory (Fermilab), reported the findings in Science1 on 7 April.
The measurement “is extremely exciting and a truly monumental result in our field”, says Florencia Canelli, an experimental particle physicist at the University of Zurich, Switzerland. If it is confirmed by other experiments, it could be the first major breach in the standard model of particle physics, a theory that has been spectacularly successful since it was introduced in the 1970s. The standard model is known to be incomplete, however, and any hint of its failing could point the way to its replacement, and to the existence of new elementary particles. “We believe there is a strong clue in this particular measurement about what nature might have in store for us,” says Ashutosh Kotwal, an experimental particle physicist at Duke University in Durham, North Carolina, who led the CDF study.
Some physicists strike a note of caution. Generating a W boson mass measurement from experimental data is famously complex. Although the work is impressive, “I would be cautious to interpret the significant difference to the standard model as a sign of new physics,” says Matthias Schott, a physicist at the Johannes Gutenberg University Mainz in Germany, who works on the ATLAS experiment at CERN, Europe’s particle-physics lab near Geneva, Switzerland. Physicists should prioritize working out why the value differs from the other recent results, he says.
Overweight particle
Since its discovery in 1983, experiments have calculated the W boson to weigh as much as 85 protons. But its exact mass has been challenging to quantify: the first experimental estimate had error margins of 5% or more. “The measurement of the W boson mass is arguably the single most challenging parameter to measure in our field,” says Mika Vesterinen, a particle physicist at the University of Warwick, UK, who works on this measurement at CERN’s LHCb experiment.
With its cousin, the Z boson, the W is involved in most types of nuclear reactions, including the fusion that powers the Sun. The W and Z bosons carry the weak nuclear force — one of the four fundamental forces of nature — similar to how every electromagnetic interaction involves the exchange of photons.
Colliders produce W bosons by smashing together particles at high energies. Experiments typically detect them through their decay into either a kind of electron or its heavier cousin, the muon, plus a neutrino. The neutrino escapes the detector without a trace, whereas the electron or muon leave conspicuous tracks.
In the decay, most of the W’s original mass transforms into the energy of the new particles. If physicists could measure that energy and the path of all the decay particles, they could immediately calculate the mass of the W that produced them. But without being able to track the neutrino, they can’t say for sure which portion of the electron or muon’s energy comes from the W’s mass and which comes from its momentum. This makes the measurement “notoriously difficult”, Vesterinen says. “You try to construct the mass when you only see half of the decay.”
Old experiment, new tricks
In the latest work, Kotwal and his collaborators aimed to make the most precise measurement ever of the W’s mass. The data had all been collected by 2011, when Fermilab’s Tevatron — a 6-kilometre-long circular machine that collided protons with antiprotons and which was once the world’s most powerful accelerator — shut down. But the latest measurement would not have been possible back then, says Kotwal. Instead, it is the result of a steady improvement of techniques in data analysis, as well as the particle-physics community’s improved understanding of how protons and antiprotons behave in collisions. “Many of the techniques to achieve that kind of precision we had not even learned about by 2012.”
The team looked at roughly 4 million W bosons produced inside the CDF detector produced 2002 and 2011 — a data set fourfold-larger than they had used in a first measurement in 20122. They calculated the energy of each decay electron by measuring how its trajectory bent in a magnetic field. One painstaking advance over the past decade was to improve the resolution of the trajectories from roughly 150 microns to less than 30 microns, says Kotwal.
After mapping the distribution of the electrons’ energies, the team calculated the boson mass that best fit the data. That was 80,433 megaelectronvolts (MeV), with an error margin of just 9.4 MeV.
The results joins the clutch of W mass measurements produced by each leading particle collider worldwide; the precision has improved since the particle’s discovery at CERN. Researchers working on CDF and DZero, the other major detector at the Tevatron, had published earlier, less-precise measurements of the W mass2,3. The ATLAS and LHCb experiments at CERN’s Large Hadron Collider, the world’s most powerful accelerator, also published their own values4,5.
The latest CDF result claims to double the precision of the previous record holder, the ATLAS measurement of 80,370 MeV with a margin of ±19. But the result disagrees with several previous measurements, which were all compatible with the best theoretical prediction of 80,357 MeV. ATLAS and LHCb are updating their W boson measurements and within a few years they should be able to reach the precision of the CDF result, says Vesterinen, and could confirm or challenge it.
A difference between the experiments lies in how the teams model the W boson’s production, which affects calculations of its mass. LHC physicists have previously highlighted faults with the program used by CDF, called Resbos; an improved iteration exists. But Kotwal says that CDF researchers picked the original technique long in advance and it would have been wrong to change techniques to make the result converge with theory.
Harbinger of theory woes
If the mass result stands, theorists will have a field day. Theoretical physicists have produced a plethora of hypothetical extensions or reforms of the standard model, and in many cases those also predict a different mass of the W boson compared with the vanilla theory. “The mass of the W is the perfect place to look for new physics and deviations from the standard model,” says Sven Heinemeyer, a theoretician at the Physics Institute of Cantabria in Spain.
Heinemeyer’s favourite explanation for the discrepancy is supersymmetry, a model that predicts the existence of a heavier partner for each standard-model particle. Such particles could continuously pop in and out of the vacuum surrounding a W particle, making it heftier.
Other possible explanations relate to the Higgs particle, another boson, which was discovered by the LHC in 2012. If the Higgs’s properties differ from those currently theorized — for example, if it is a composite particle rather than an elementary one, or if multiple versions of it exist — this would affect the W mass.
Another major LHC experiment, called CMS, is working on its own measurement of the W boson’s mass, says Canelli, a leading researcher in the CMS collaboration (Canelli is also a member of the CDF collaboration, but she was not involved in generating the latest result to avoid conflicts of interest). “It is one of the most important measurements from our physics programme.”
[“source=nature”]